Physics of snakeskin
sheds light on sidewinding
Discovery may aid robot locomotion
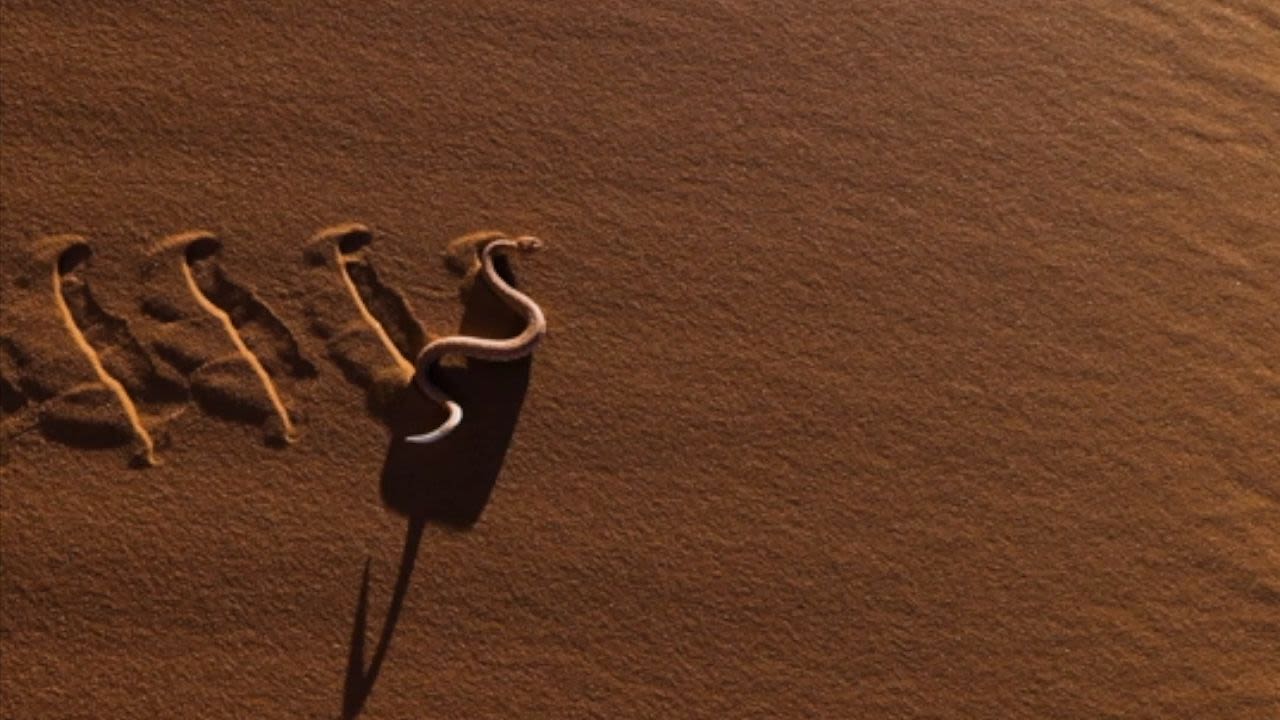
Most snakes get from A to B by bending their bodies into S-shapes and slithering forward headfirst. A few species, however — found in the deserts of North America, Africa and the Middle East — have an odder way of getting around. Known as “sidewinders,” these snakes lead with their mid-sections instead of their heads, slinking sideways across loose sand.
Scientists took a microscopic look at the skin of sidewinders to see if it plays a role in their unique method of movement. They discovered that sidewinders’ bellies are studded with tiny pits and have few, if any, of the tiny spikes found on the bellies of other snakes.
The Proceedings of the National Academy of Sciences (PNAS) published the discovery, which includes a mathematical model linking these distinct structures to function.
“The specialized locomotion of sidewinders evolved independently in different species in different parts of the world, suggesting that sidewinding is a good solution to a problem,” says Jennifer Rieser, assistant professor of physics at Emory University and a first author of the study. “Understanding how and why this example of convergent evolution works may allow us to adapt it for our own needs, such as building robots that can move in challenging environments.”
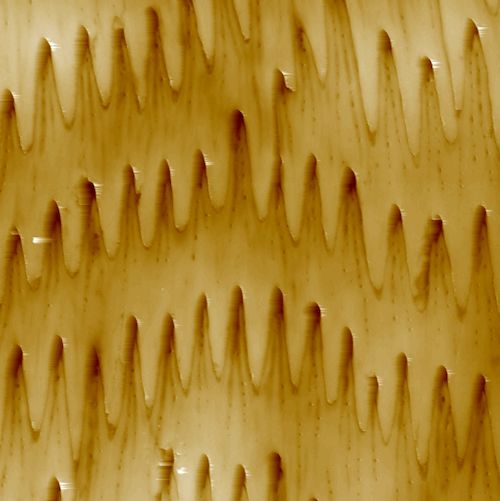
The Mexican lance-headed rattlesnake (Crotalus polystictus) moves by slithering forward. A micrograph from the study, above, of the skin of its belly reveals spikes that are normally invisible to the eye. The micrograph image shows a surface 20 microns wide, or about a third of the width of a human hair. (Tai-De Li)
The Mexican lance-headed rattlesnake (Crotalus polystictus) moves by slithering forward. A micrograph from the study, above, of the skin of its belly reveals spikes that are normally invisible to the eye. The micrograph image shows a surface 20 microns wide, or about a third of the width of a human hair. (Tai-De Li)
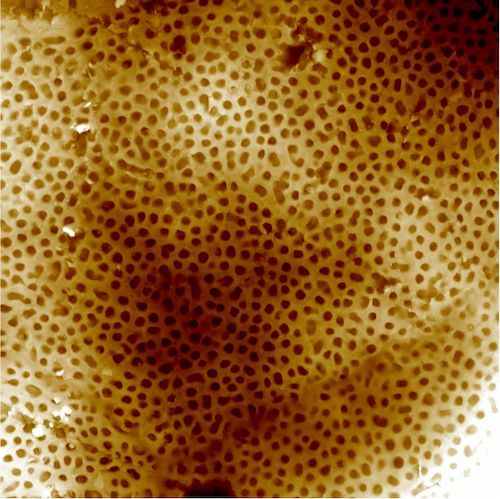
A micrograph of the skin of the Saharan sand viper (Cerastes vipera), a sidewinder, reveals that its belly is studded with pits instead of spikes. Image shows a surface about 20 microns wide. (Tai-De Li)
A micrograph of the skin of the Saharan sand viper (Cerastes vipera), a sidewinder, reveals that its belly is studded with pits instead of spikes. Image shows a surface about 20 microns wide. (Tai-De Li)
Co-authors of the paper include Joseph Mendelson, a herpetologist and the director of research at Zoo Atlanta; evolutionary biologist Jessica Tingle (University of California, Riverside); and physicists Daniel Goldman (Georgia Tech) and co-first author Tai-De Li (City University of New York).
Rieser’s research interests bring together the physics of soft matter — flowable materials like sand — and organismal biology. She studies how animals’ surfaces interact with the flowable materials in their environments to get around. Insights from her research may lead to improvements in human technology.
Snakes, and other limbless locomotors, are particularly interesting to Rieser. “Even though snakes have a relatively simple body plan, they are able to navigate a variety of habitats successfully,” she says. Their long, flexible bodies are inspiring work on “snake” robots for everything from surgical procedures to search-and-rescue missions in collapsed buildings, she adds.
In a previous paper, Rieser and colleagues found that designing robots to move in serpentine ways may help them to avoid catastrophe when they collide with objects in their path.
Sidewinders offered her a chance to dig further into how nature has evolved ways to move across loose sand and other soft matter.
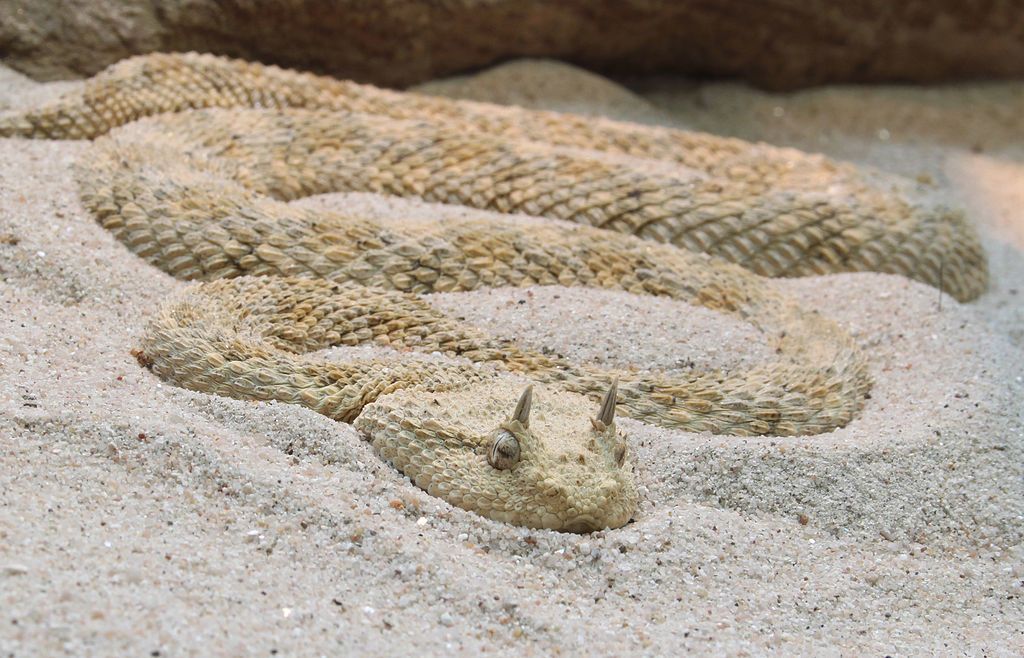

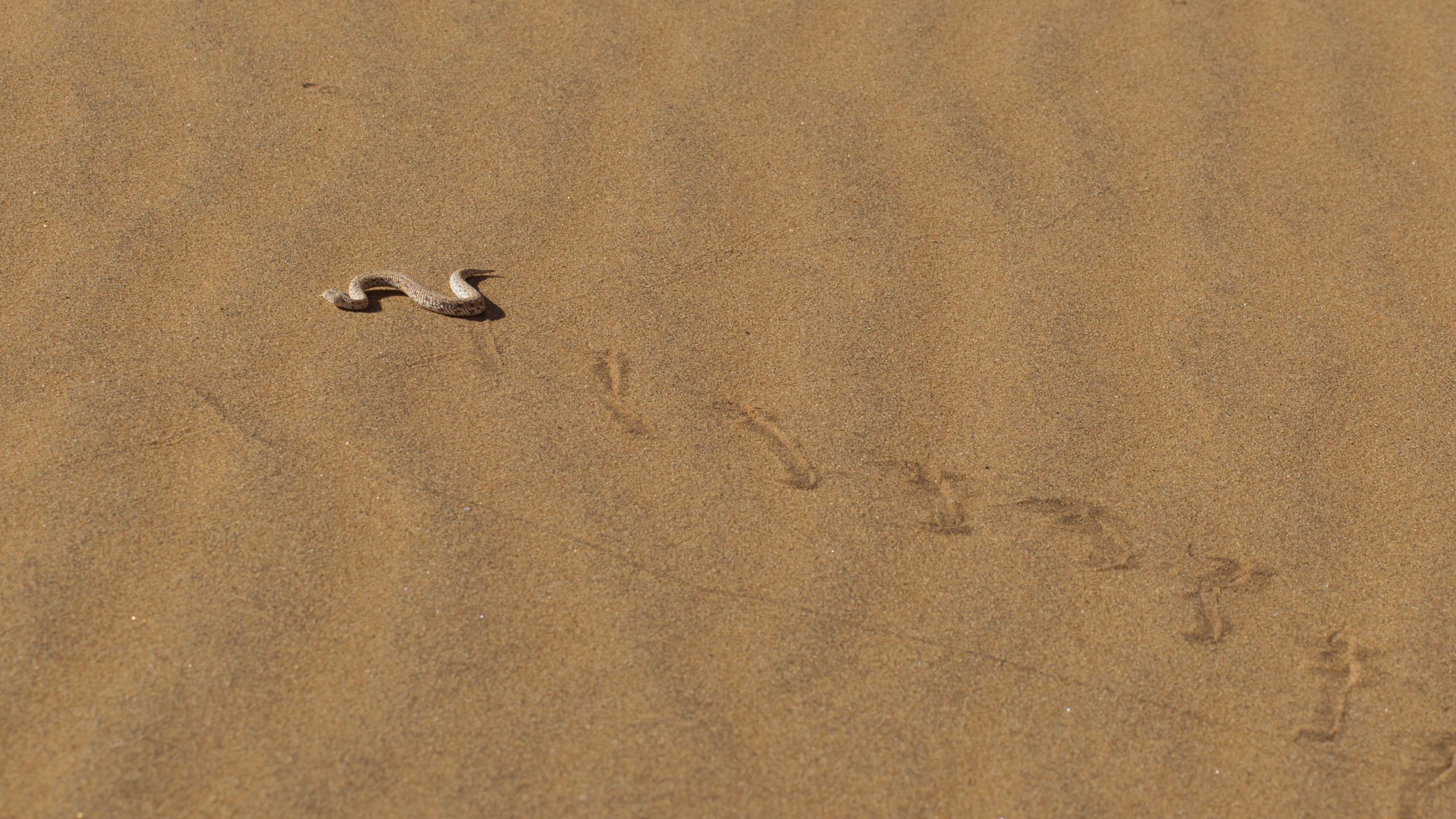
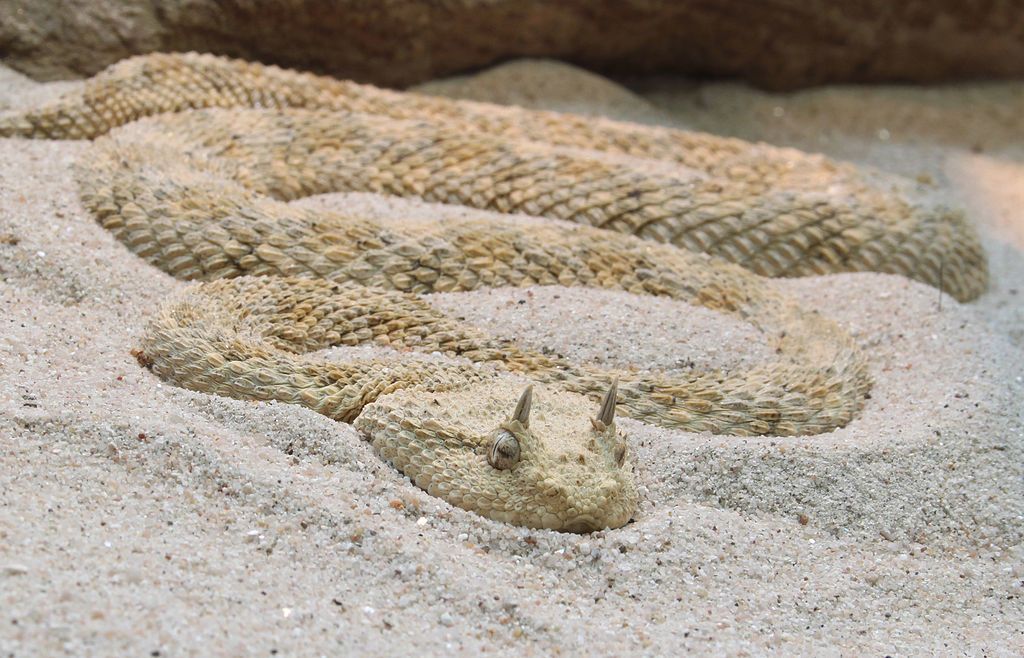
A Saharan horned viper (Cerastes cerastes) is a species of sidewinder native to the deserts of northern Africa and parts of the Arabian peninsula. Sidewinders in this region have had longer to adapt to a desert environment. (Wikipedia, Holger Krisp)
A Saharan horned viper (Cerastes cerastes) is a species of sidewinder native to the deserts of northern Africa and parts of the Arabian peninsula. Sidewinders in this region have had longer to adapt to a desert environment. (Wikipedia, Holger Krisp)

The sidewinder rattlesnake (Crotalus cerastes), found in the southwestern United States and northwestern Mexico, has had less time evolutionarily to adapt to a sandy environment, since American sandy deserts are much younger than those in Africa. (Wikipedia: Tigerhawkvok)
The sidewinder rattlesnake (Crotalus cerastes), found in the southwestern United States and northwestern Mexico, has had less time evolutionarily to adapt to a sandy environment, since American sandy deserts are much younger than those in Africa. (Wikipedia: Tigerhawkvok)
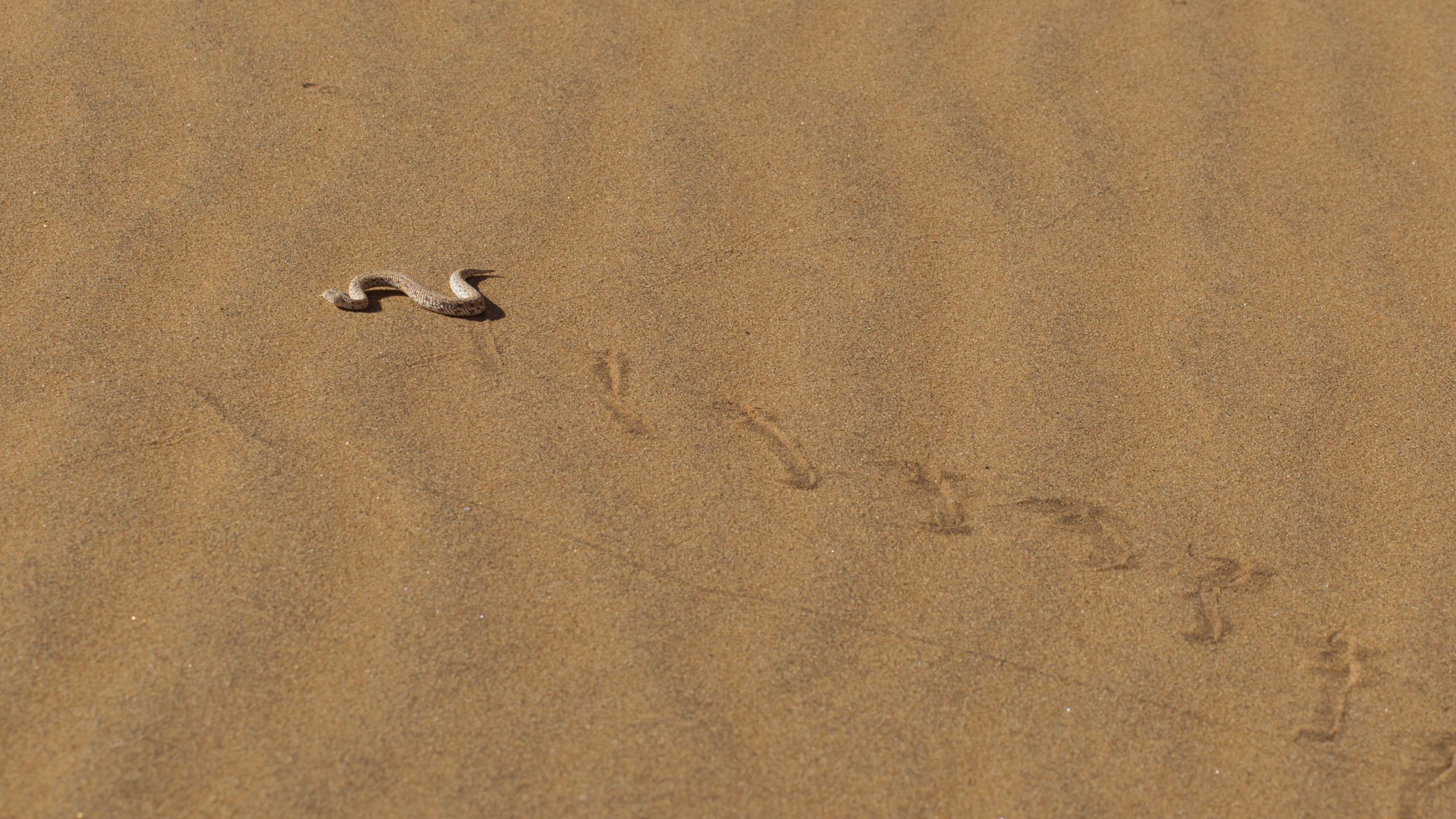
A sidewinder leaves distinctive tracks across a sand dune. (Getty Images)
A sidewinder leaves distinctive tracks across a sand dune. (Getty Images)
Most snakes tend to keep their bellies largely in contact with the ground as they slide forward, bending their bodies from their heads to their tails. A sidewinder, however, lifts its midsection off the ground, shifting it in a sideways direction.
Previous studies have hypothesized that sidewinding may allow a snake to move better on sandy slopes. “The thought is that sidewinders spread out the forces that their bodies impart to the ground as they move so that they don’t cause a sand dune to avalanche as they move across it,” Rieser explains.
For the current paper, Rieser and her colleagues investigated whether sidewinders’ skin might also play a role in their unique movement style.
They focused on three species of sidewinders, all of them vipers, in residence at zoos: The sidewinder rattlesnake (Crotalus cerastes), found in the deserts of the Southwestern United States and northern Mexico; and the Saharan horned viper (Cerastes cerastes) and the Saharan sand viper (Cerastes vipera), both from the deserts of north Africa.
Skins shed from the sidewinders were collected and scanned with atomic force microscopy, a technique that provides resolution at the atomic level, on the order of fractions of a nanometer. For comparison, they also scanned snake skins shed from non-sidewinders.
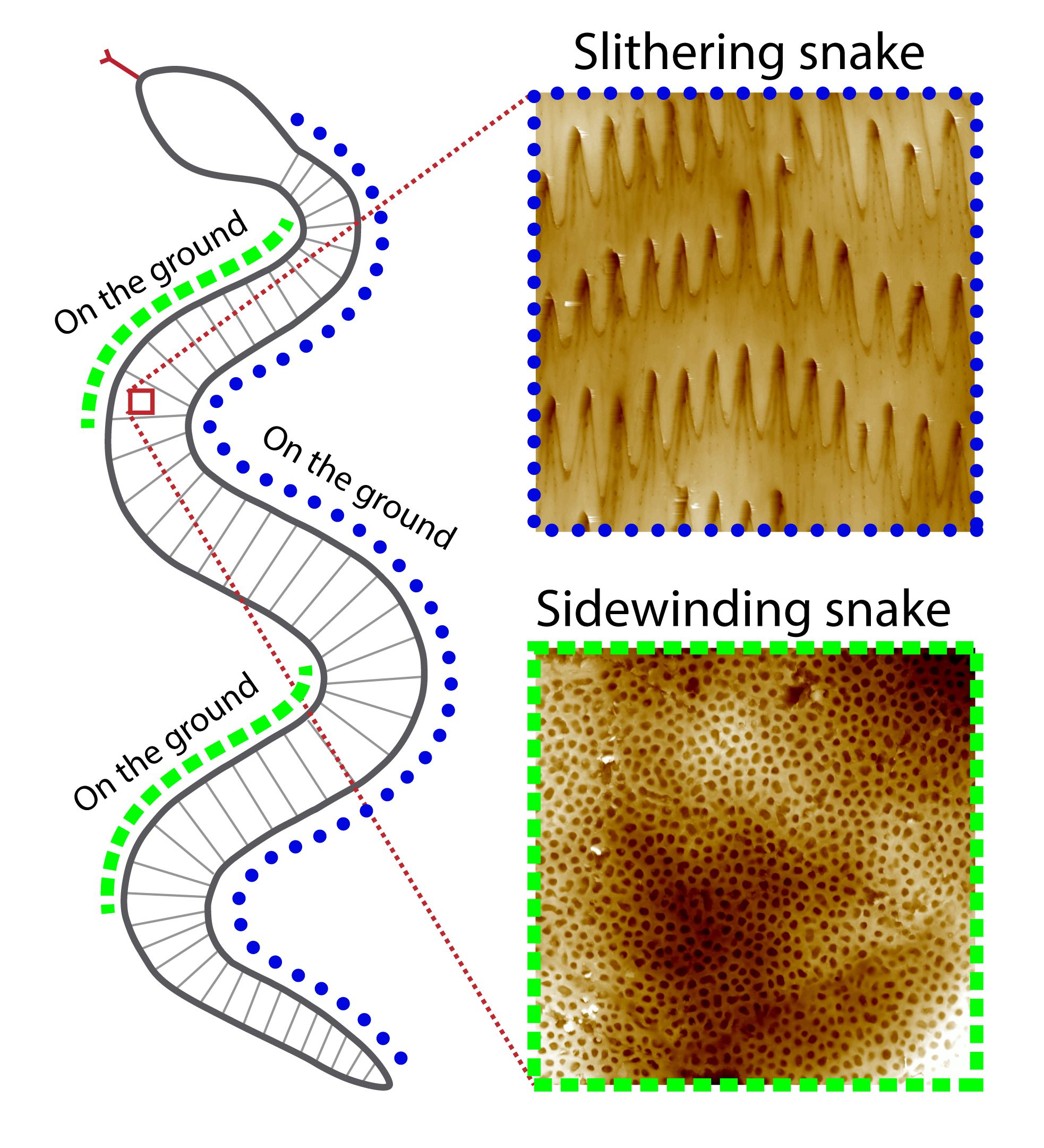
Graphic shows how snakes that slither forward and sidewinders use their bodies differently for locomotion, along with microscopic differences in the skin of their bellies. (Jennifer Rieser)
Graphic shows how snakes that slither forward and sidewinders use their bodies differently for locomotion, along with microscopic differences in the skin of their bellies. (Jennifer Rieser)
As expected, the microscopy revealed tiny, head-to-tail pointing spikes on the skin of the non-sidewinders. Previous research had identified these micro spikes on a variety of other slithering snakes.
The current study, however, found that the skin of sidewinders is different. The two African sidewinders had micro pits on their bellies and no spikes. The skin of the sidewinder rattlesnake was also studded with tiny pits, along with a few, much smaller, spikes — although far fewer spikes than those of the slithering snakes.
The researchers created a mathematical model to test how these different structures affect frictional interactions with a surface. The model showed that head-to-tail pointing spikes enhance the speed and distance of forward undulation but are detrimental to sidewinding.
“You can think about it like the ridges on corduroy material,” Rieser says. “When you run your fingers along corduroy in the same direction as the ridges there is less friction than when you slide your fingers across the ridges.”
The model also showed that the uniform, non-directional structure of the round pits enhanced sidewinding, but was not as efficient as spikes for forward undulation.
The research provides snapshots at different points in time of convergent evolution — when different species independently evolve similar traits as a result of having to adapt to similar environments.
Rieser notes that American sandy deserts are much younger than those in Africa. The Mojave of North America accumulated sand about 20,000 years ago while sandy conditions appeared in the Sahara region at least seven million years ago.
“That may explain why the sidewinder rattlesnake still has a few micro spikes left on its belly,” she says. “It has not had as much time to evolve specialized locomotion for a sandy environment as the two African species, that have already lost all of their spikes.”
Engineers may also want to adapt their robot designs accordingly, Rieser adds. “Depending on what type of surface you need a robot to move on,” she says, “you may want to consider designing its surface to have a particular texture to enhance its movement.”
Story and design by Carol Clark
To learn more:
Emory Department of Physics
Media inquiries: Carol Clark, 404-727-0501
carol.clark@emory.edu
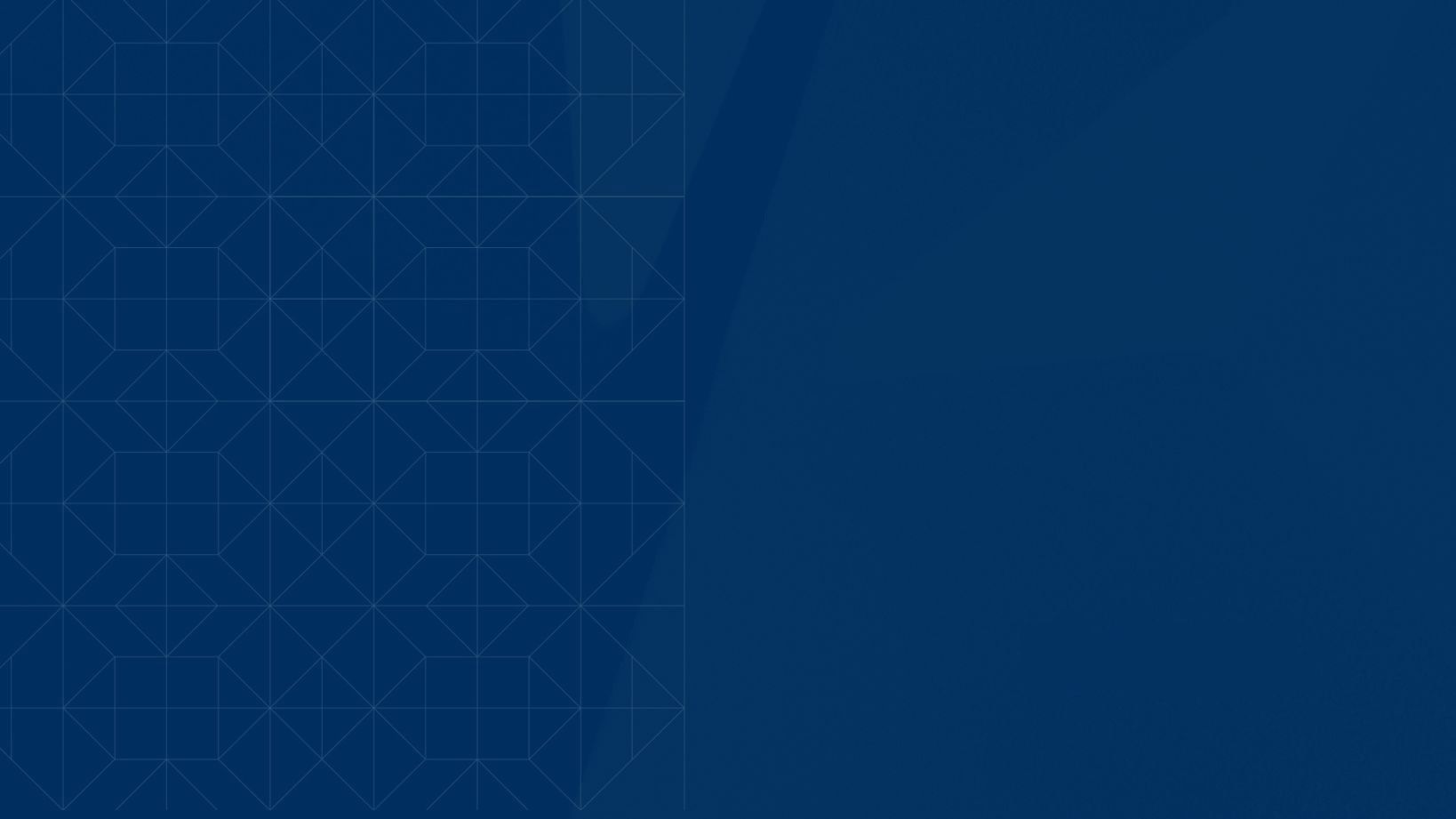